The Catalysis and Energy Laboratory is part of the research group Nanoengineering of Materials Applied to Energy (NEMEN) at UPC. One of its main activities is the development of catalysts and catalytic reactors for hydrogen-related technologies. NEMEN is part of the Institute of Energy Technologies and the Department of Chemical Engineering of UPC.
One of the main goals to fully develop nuclear fusion reactors is the recovery of hydrogen isotopes and its separation from the impurities generated in the main nuclear fusion reactor. The use of Pd-based membranes is widespread, but the poisoning of those membranes by carbon monoxide (CO) or methane (CH4), which are present as impurities in nuclear fusion reactors, drastically decreases their lifetime. Therefore, membranes must be protected to increase their durability and high performance. In this sense, our main goal, which contributes the objective of task 2.4 of FusionCAT project, is to prepare a multicomponent catalyst to shelter the membrane by decomposing and/or transforming the impurities without consuming hydrogen and at the same time allowing the fully performance of the Pd-based membrane.
The selection and development of a multicomponent catalyst is a challenge as the main reactions involve during the catalytic process to eliminate all the impurities take place at different reaction conditions, such as temperature and pressure. Therefore, taking into account the main catalysts found in the literature, different formulations of catalysts have been investigated.
In chemistry, a catalyst support is the material, commonly a solid, with a high surface area, to which the catalyst is affixed. The support may be catalytically inert, but frequently it contributes to the overall catalytic activity. Moreover, the activity of a catalyst is boosted by atoms present at the accessible surface of the material. Therefore, it is not only important to find the most appropriate support for each catalyst, but also to maximize the specific surface area. The main supports analyzed are those based on ceria (CeO2), alumina (Al2O3), zirconia (ZrO2) and lanthanum oxide (La2O3) and Ni, Fe and Rh as active phases. The catalysts prepared, the amount or weight percentage (%wt) of active phase and the precursors used are summarized in table 1.
Catalyst | Active phase (% wt.) | Support precursors | Active phase precursors | |
---|---|---|---|---|
1 | NiFe/CeLaOx | 15% Ni, 3% Fe | Ce(NO3)3, La(NO3)3 | Fe(NO3)3, Ni(NO3)2 |
2 | NiFe/AlCeZrLaOx | 15% Ni, 3% Fe | CeCl3, ZrOCl2, Al(OH)3, La(NO3)3 | Fe(NO3)3, Ni(NO3)2 |
3 | NiFeRh/AlCeZrLaOx | 14,6% Ni, 2,9% Fe, 1,5% Rh | Fe(NO3)3, Ni(NO3)2, RhCl3 | |
4 | Rh/AlCeZrLaOx | 1,5% Rh | CeCl3, ZrOCl2, Al(OH)3, La(NO3)3 | RhCl3 |
Table 1: Catalysts prepared.
In order to test the viability of the different catalysts prepared under different temperature and pressure conditions, a reaction system was designed, Figure 1. The system consists of four gas lines (CO, O2, H2 and CH4) connected to mass controllers and a liquid line (H2O) connected to a high performance liquid chromatography (HPLC) injection bomb. Each line is heated and connected to a non-return valve prior to the reactor, which is placed in a furnace regulated with a temperature controller (± 0,1 °C) equipped with a thermocouple. A filter, a pressure regulating valve and a manometer are located at the outlet of the reactor. Next, a condenser and a gas chromatograph are connected to the outlet to measure the composition of the mixture online every 5 minutes, during the course of the experiment, and a volumetric gas flow meter. The catalytic bed has been kept uniform in all experiments (0.6 cm3) by dilution with silicon carbide.
Figure 1: Schema of the reaction system used.
Samples have been analysed before and after reaction by X-ray diffraction (XRD) and Raman spectroscopy to determine the crystalline phases present. X-ray Photoelectron Spectroscopy (XPS) has also been used to determine the oxidation states and the surrounding environment of all the elements present in each catalyst. Finally, the catalytic performance of the different catalyst has been analysed. Figure 2 shows the evolution of the CO conversion as a function of the catalyst used and of the temperature. Results show that the most promising catalysts are those based on ceria-alumina-zirconia-lanthanum oxide support with Ni/Fe or Ni/Fe/Rh as active phase with a 100 % conversion of CO in a wide range of temperatures.
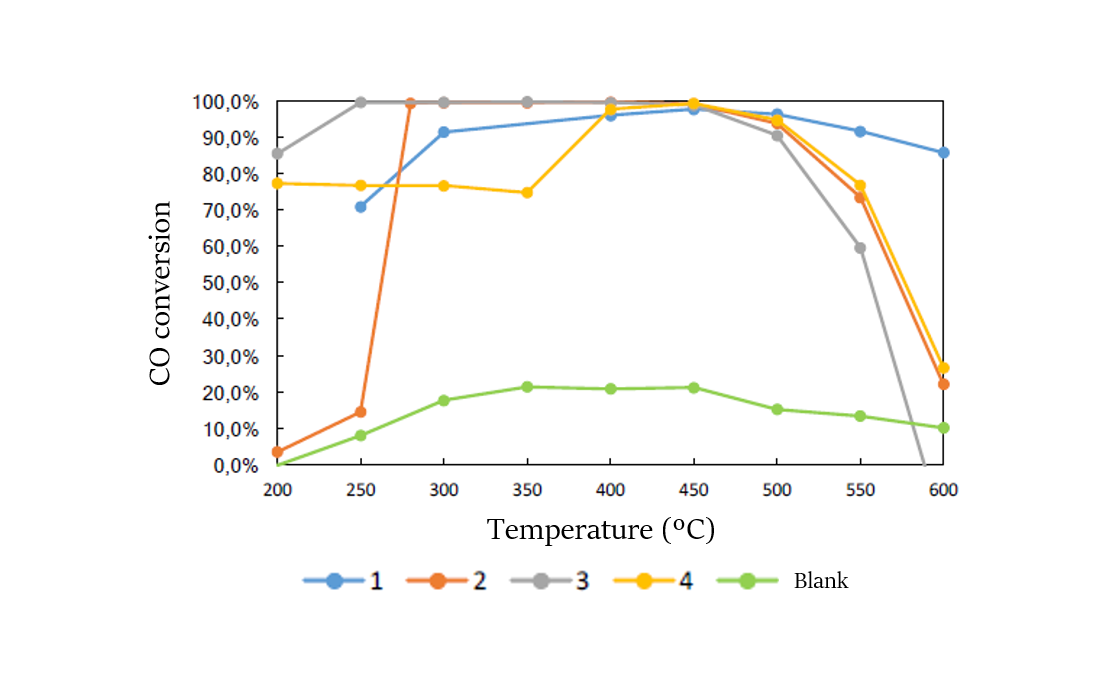
Figure 2. Evolution of the CO conversion as a function of the catalyst and of the temperature.
The next step now is to develop a catalytic membrane reactor (CMR) and to integrate the studied catalysts in a pelletized form with the Pd-alloy membrane, and to study the performance of the final device under different pressure and temperature values.